< Back to Newsroom
Powering Up: The Technologies Providing Energy Storage’s “Flex” on the Grid
Energy storage has grown dramatically in the last decade, both in terms of solutions available and capacity already installed. In this edition of Powering Up, we’ll explain how today’s energy storage technologies provide both the grid and large-scale energy users more flexibility in meeting their needs.
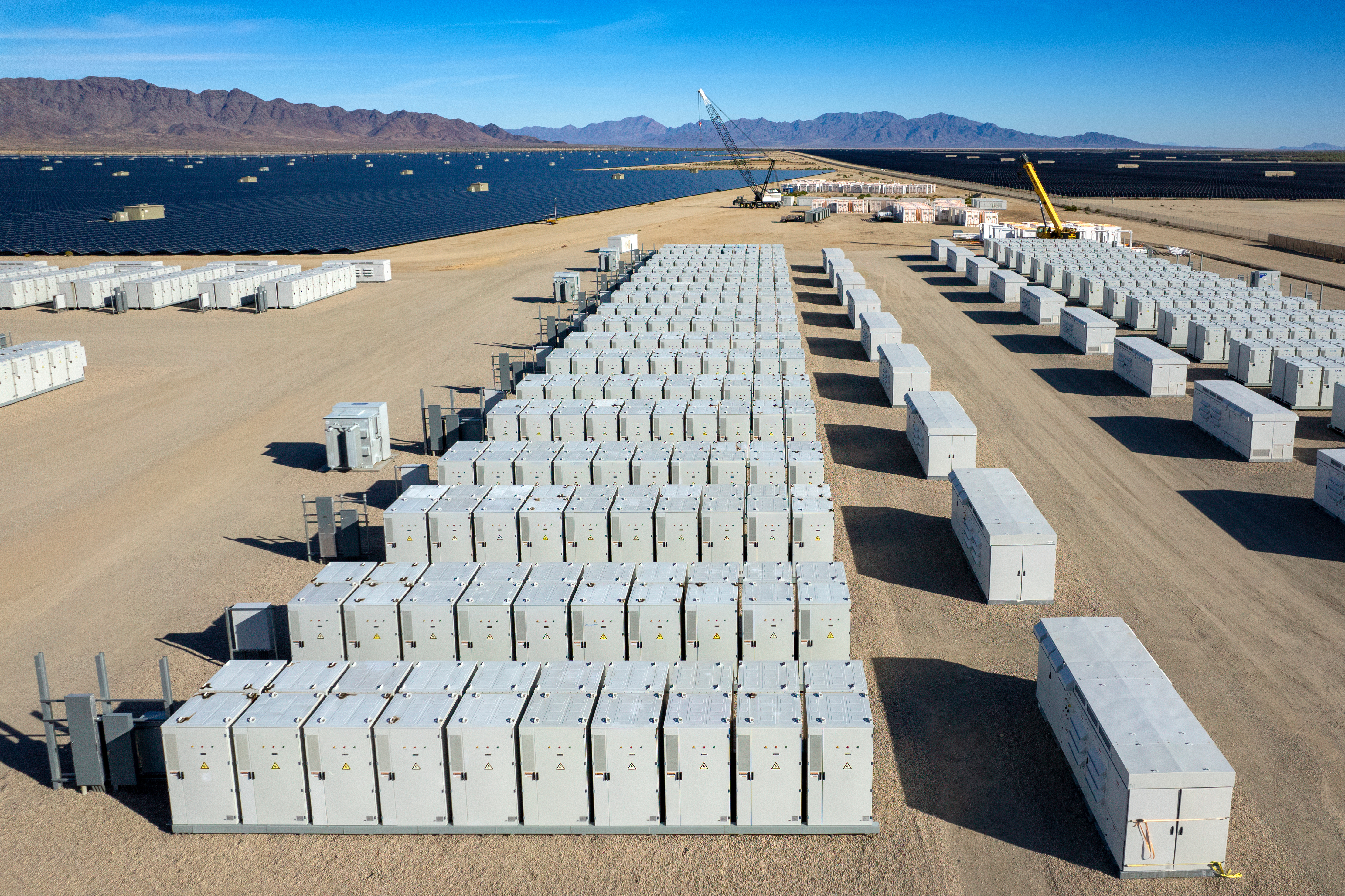
May 14 2025 | 10 min read
From tech leaders to consumer brands, enterprise customers have set ambitious targets for incorporating more renewable energy into their daily operations. While some energy sources like hydropower and nuclear can operate 24/7, solar and wind power are intermittent. If too much solar or wind power is generated, that power may have to be curtailed to avoid overloading power lines. That’s why energy storage – particularly in the form of large-scale battery projects – has become one of the fastest-growing parts of the energy landscape, making it possible to store energy generated by renewables and other generation and deploying it at times when it’s most needed.
In our last post, we looked at the “jobs to be done” by energy storage. In this one, we’re looking at the variety of technologies that are doing those jobs, how quickly they’ve gained market share, and most importantly, what role they play in helping make electricity grids more flexible and reliable and bringing more clean energy into our overall resource mix.
Credit: U.S. Department of Energy
The old standard: pumped hydroelectric storage
Pumped hydroelectric energy storage (PHES) is currently the most common long-duration — typically meaning 8+ hours — energy storage method in the world.
PHES uses two reservoirs at different elevations to act as a type of hydrological “battery.” When solar or other renewable sources are peaking in energy production, water is pumped from the lower reservoir to the higher one. As renewable production wanes—for instance, when the sun goes down—a controlled flow of water is released from the higher reservoir to flow through turbines that produce electricity. 70-85% of each kWh used to pump water is recovered during the production phase.
PHES assets are typically designed to provide anywhere from 6 to 20 hours of energy storage but can be run at varying volumes to extend that time at lower output. While PHES use is slightly declining with the rapid adoption and ease of deployment with advanced lithium-ion battery storage and other options, it still represents a large portion of utility-scale storage capacity.
Pros:
- It’s easy to control the amount of power generated with water flows.
- PHES smooths energy fluctuations from intermittent renewable supplies.
- It can provide up to 20+ hours of stored energy (much more than today’s larger battery installations).
- Reservoirs can mitigate the effects of flooding or droughts.
- Ample sites with preexisting global paired reservoirs across the globe could be employed without disrupting river flows.
- Land and water use are low, and water can be recirculated within the reservoir system.
- PHES uses significantly less water fossil-fuel applications.
Cons:
- Like traditional hydropower dams, the water flows to and from PHES can disrupt riverine environments.
- Its storage capacity is greater than lithium-ion batteries, but isn’t useful for long-term applications of days or weeks.
Building up faster than Minecraft: battery energy storage systems
Battery-based energy storage systems are one of the fastest growing energy storage options, with lithium-ion (Li-ion) chemistries considered the industry standard. Today, the most common is lithium-ion phosphate (LFP), which started predominantly in electric vehicles, and was adopted more broadly for stationary energy storage because of its lower risk of safety incidents. They’re largely paired with solar and wind farms, and are integral to EV infrastructure.
Today’s battery chemistries, like LFP or nickel-manganese-cobalt (NMC), are currently the most energy-dense battery energy storage options, and are easily scalable without much alteration of existing infrastructure. Energy density is projected to improve with the development of different design options, such as silicon or lithium-metal anodes and solid-state electrolytes.
There’s been intense growth and investment in the BESS market, with 90% of lithium-ion battery demand attributed to the energy sector. The U.S. market is expected to double by 2030, with 300 utility-scale battery storage projects expected to be developed by 2025. Further, as a result of incentives created under the Inflation Reduction Act and tariffs levied on some imported battery sources, U.S. manufacturing of battery packs and systems is ramping up to create more domestically-produced supply.
Battery pros:
- Batteries are becoming cheaper, and while China used to dominate the market, increasingly the USA and countries in the EU and Southeast Asia are growing their manufacturing capabilities for energy storage.
- Lithium is becoming more available as the U.S. explores deposits across the country.
- Li-ion batteries paired with renewables can offer better alternatives for lead-acid batteries and expensive diesel generators as back-up power for critical infrastructure, like hospitals, groceries, and data centers, even in remote areas with industries that are completely off-grid
- Integrating batteries with rooftop solar can significantly reduce energy costs.
- Because of its compact footprint, battery storage can be easily sited alongside PV installations or EV charging facilities to maximize performance of those technologies.
- Lithium-based chemistries are well-understood, mature technology with robust supply chains and capacity available.
- New developments in sodium-based batteries will soon provide an alternative to lithium-based chemistries, based on materials that are far easier to source.
Cons:
- The United States remains reliant on lithium for a majority of battery storage applications, and currently depends on China for refined lithium and a range of other metals currently used in battery manufacturing.
- Battery storage is primarily used for “shorter” duration storage, typically <1 hour up to 6-hour use cases, though recently battery systems have been selected for 8-hour duration uses in California. Additional battery chemistries, like flow batteries or iron-air, are capable of providing much longer duration, though with tradeoffs on cost and scale.
Quickly working up the maturity curve: long-duration energy storage
Today, long-duration energy storage (LDES) refers to energy storage with a capacity of 6+ hours. These technologies have the potential to solve energy storage issues for off-grid applications where fossil-fuel use is high and firm renewables are needed to smooth out the power supply. PHES was the first example of LDES, though other technologies in development can provide greater storage capacity and duration. A 2024 report from the LDES Council estimates that LDES could reduce industrial GHG emissions by 65% if paired with renewables.
LDES comes in four forms: mechanical, chemical, thermal, and electrochemical.
Mechanical energy storage technologies combine machinery with heat, water, or air for long-duration storage. While PHES is the most prevalent mechanical storage type, compressed air energy storage (CAES), which stores compressed air underground for later release to power turbines, is another example.
Hydrogen fuel cells, a chemical-based LDES solution, have been in use since NASA deployed them in the Gemini spacecraft. They work by combining hydrogen and oxygen within a fuel cell, producing electricity through the splitting of hydrogen molecules and water as a byproduct. Hydrogen can be sourced from water or fossil fuels.
Thermal energy storage options, like thermal “batteries” that store energy as heat or steam-based heat pumps can deliver utility-scale storage by storing heat and releasing steam to turn turbines.
Adoption of LDES technology will eventually be key to supporting broad renewable energy use on the grid, particularly within industries like mining and manufacturing operations with intensive operational needs. It can help industries like steel and cement manufacturing, which traditionally command vast amounts of fossil fuel use with high energy and thermal needs, shift to electrified production.
LDES pros:
- LDES can help companies and manufacturing industries answer electric and thermal demands while meeting sustainability targets.
- They help extend the life of industrial infrastructure
- They’re less polluting and support community health.
- Customers may pay premiums for products produced with green energy.
- They reduce reliance on other expensive fuels, such as diesel, for backup power.
- They’re now cheaper than Li-ion batteries for use over 8+ hours.
Cons:
- Some LDES technology is still in the experimental phase.
- LDES requires a large investment, which has caused some hesitation in adopting their use.
What’s the right solution?
Battery storage has gained the most prominence for grid and onsite energy storage applications for its small footprint, maturity of technology, modularity of design and ability to be sited nearly anywhere with a grid connection. Moreover, the speed of deployment is currently outpacing either of the other technology sets – though availability of key components like transformers can affect that.
However, LDES technologies and especially thermal energy storage are offering paths to reducing carbon emissions and fuel use in industries where batteries can’t provide enough duration or where shifting to electrification isn’t feasible.
TL;DR – there’s no one-size-fits-all storage option — technology choice comes down the needs, usually in terms of services each can provide, deployment speed, project cost and longevity of the asset.